By Jacob Jerome, RJD Graduate Student and Intern
There have been numerous studies that focus on the alterations that climate change can have on the marine environment and how those alterations affect corals. In the marine science field coral bleaching and the disappearance of coral reefs is widely discussed. One of the primary debates centers around whether or not it is too late to save coral reefs. But is this doom and gloom viewpoint how we should be looking at this situation? Many scientists argue that there is still hope for coral reefs.
It is important to first understand the threats that climate change pose to corals. There are two main threats: a rise in ocean temperatures and a lowering of the ocean’s pH, a process known as ocean acidification.
Higher temperatures stress corals and cause them to lose their symbiotic algae, or zooxanthellae (NOAA,2011). These symbiotic algae are what give corals their color and without them the corals turn white, an event known as coral bleaching. This bleaching can have several negative impacts on the coral polyps. Corals and their symbiotic algae have what is called a mutualistic symbiotic relationship; this is a relationship where both species benefit from interacting with one another. Corals provide their symbiotic algae with a protected environment and compounds they need for photosynthesis. The symbiotic algae, in return, provide corals with the products of photosynthesis, a suite of compounds that provide food for the corals and aid in the production of calcium carbonate. Although still alive, by losing their symbiotic algae, corals experience increased stress and are more prone to disease (NOAA, 2011).
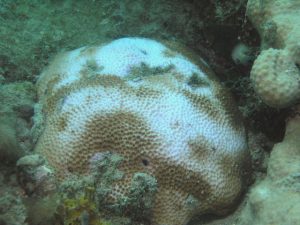
A clear depiction of coral bleaching (Joe Bartoszek 2010/Marine Photobank)
Ocean acidification occurs due to the overwhelming amount of carbon dioxide that is absorbed into the ocean from the Earth’s atmosphere. When carbon dioxide is absorbed into the water, the pH of the water decreases and the water becomes more acidic. Low pH waters limit the rate at which corals can produce calcium carbonate and also increase the rate at which calcium carbonate dissolves (Andersson et al., 2014). Corals use calcium carbonate to build their hard exoskeleton. If corals are not able to produce calcium carbonate quicker than the rate at which it dissolves, they cannot grow.
Knowing these threats, many assume that corals have little hope for surviving through the end of this century. According to the Status of Coral Reefs of the World: 2008, 19 percent of the world’s coral reefs are gone or cannot recover, 15 percent are seriously threatened, and 20 percent are under the threat of loss within the next 20 to 40 years. So, is it too late for corals? Are these threats too great for us to effectively manage them? New scientific research indicates that not all corals are quite ready to give up.

A table summarizing the status of the world’s coral reefs in 2008 (Wilkinson, C. 2008)
Just last year, Australian scientists discovered that coral animals alone are able to produce dimethylsulphoniopropionate (DMSP), a sulphur-based molecule with properties that can provide protection on a cellular level to corals in times of heat stress (Raina et al., 2013). This was the first time that an animal had been discovered to produce DMSP. They also found that corals increased their production of DMSP when subjected to higher water temperatures (Raina et al., 2013). This new information illustrates that corals, even without their symbiotic algae, can “fight” against temperature shifts. While this does not mean that corals can entirely defend themselves against rising temperatures, it does indicate an ability to adapt, to an extent, to these changes.
In addition, a study in the Cayman Islands revealed that a coral reef system that suffered a 40 percent reduction in corals due to bleaching and diseases was able to recover seven years later (Manfrino et al., 2013). The corals in the Cayman Islands are known to be healthy and are afforded some protection from fishing and anchoring. This protection definitely aided in their recovery along with their isolation, a small human population, and a generally healthy ecology (Manfrino et al., 2013). Nonetheless, the Cayman Islands can serve as an example of what can happen when reef management is taken seriously.
In Palau, something remarkable has been discovered. By taking water samples from 9 different locations that stretched from open ocean, across a barrier reef, and into a lagoon and bays, scientists discovered that the sea water became increasingly acidic as they moved toward land (Shamberger et al., 2014). What was even more surprising was that the level of acidity was as high as scientists had predicted for the open ocean by the end of this century. Even so, healthy and diverse coral reefs were found in these areas. In fact, the corals appeared healthier in the more acidic areas than they did in the less acidic areas (Shamberger et al., 2014). While these results are incredible, caution should be taken when interpreting them. The environment surrounding the corals of Palau might create a “perfect storm” for environmental conditions that allow the corals to survive in the acidic waters. Even so, this area has been functioning the same way for thousands of years and may have unintentionally modified the corals in that area genetically. If this is the case, those corals can essentially be put in other acidic environments and survive. This discovery could have huge implications for the survival of corals.
It is important that we do not lose sight of the fact that these new discoveries do not mean that corals are safe under ocean conditions that have resulted from climate change. It does mean, however, that there is still hope for some corals. Climate change is difficult to prevent and changing human habits can be even harder. But if we can release the myriad of other stresses that are put on corals and think about our carbon footprint, corals just might stand a chance for their beauty to be enjoyed for generations to come.
References
Andersson, A. J., Yeakel, K. L., Bates, N. R., de Putron, S. J. (2014). “Partial offsets in ocean acidification from changing coral reef biogeochemistry.” Nature Climate Change, 4(1): 56–61.
“Coral Bleaching And Ocean Acidification Are Two Climate-Related Impacts to Coral Reefs.” How Is Climate Change Affecting Coral Reefs? Ed. National Ocean Service. NOAA, 8 Dec. 2011. Web. 10 Mar. 2014. <http://floridakeys.noaa.gov/corals/climatethreat.html>.
Manfrino, C., Jacoby, C.A., Camp, E., Frazer, T.K. (2013). “A Positive Trajectory for Corals at Little Cayman Island.” PLoS ONE, 8(10): e75432.
Raina, J.B., Tapiolas, D.M., Forêt, S., Lutz, A., Abrego, D., Ceh, J., Seneca, F.O., Clode, P.L., Bourne, D.G. Willis, B.L., Motti, C.L. (2013). “DMSP biosynthesis by an animal and its role in coral thermal stress response.” Nature, 502: 677-680.
Shamberger, K. E. F. Cohen, A.L., Golbuu, Y., McCorkle, D.C., Lentz, S.J., Barkley, H.C. (2014). “Diverse coral communities in naturally acidified waters of a Western Pacific Reef.” Geophysical Research Letters, 41: 499–504.
Wilkinson, C. (2008). Status of the Coral Reefs of the World: 2008. Global Coral Reef Monitoring Network and Reef and Rainforest Research Centre, Townsville, Australia, 296p. Reefcheck.org 3/10/2014.