The Lasting Legacy of the Deepwater Horizon Oil Spill
By Delaney Reynolds, SRC intern
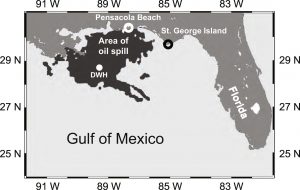
This map of how far the oil reached on the surface level of the Gulf of Mexico exhibits that the coasts of Texas, Louisiana, Mississippi, Alabama, and Florida were impacted.
(Source: Huettel, M., Overholt, W. A., Kostka, J. E., Hagan, C., Kaba, J., Wells, B., & Dudley, S. (2017, December 22). Degradation of Deepwater Horizon oil buried in a Florida beach influenced by tidal pumping. Retrieved March 13, 2018, from https://www.sciencedirect.com/science/article/ pii/S0025326X1730903)
Mankind’s use of fossil fuels as an energy source can place our natural environment at grave risk, and nowhere is that more acute than in the Gulf of Mexico. The environmental threats the Gulf region faces from petroleum production and exploration are not just those that appear in the media immediately following an oil spill or similar catastrophe, but are events that leave a lasting, often unseen legacy that stands to pollute and destroy our natural environment and the creatures that live in it for generations.
The Deepwater Horizon, British Petroleum (BP), oil spill of 2010 was the largest marine oil spill in history and polluted the Gulf for 87 days by pouring an estimated 60,000 barrels per day at its peak, and over 3.19 million barrels in total, of petroleum into the Gulf’s environment (Pallardy). The oil’s effluence rapidly spread to over 1,000 miles on the coastlines of Texas, Louisiana, Mississippi, Alabama, and Florida and while the efforts to clean up beaches and the spill itself have had some success, remnants of oil remain buried in sediments and continue to dramatically disrupt life beneath the surface (Frost).
Florida State University researchers discovered that within a week of burial, two thirds of the oil that washed ashore was retained in coastal sediments and caused a decrease in biodiversity by over 50% (Huettel). Bacterial abundance increased drastically in heavily oiled sands as the bacteria thrived off the oil and, thus, caused bacteria blooms, lowering overall oxygen content. This decrease in oxygen content, in turn, caused the decrease of biodiversity as aerobic organisms either perished or migrated to areas with a higher oxygen content. However, within three months, a resurgence in microorganisms normalized biodiversity as they restocked the coastal waters with the oxygen that aerobic organisms’ survival necessitates. Not only does this exemplify the ability of aquatic ecosystems to replenish themselves after being exposed to stressors, but it also supplies us with knowledge of the types of microorganisms that could be utilized to clean up future spills, as well as any environmental impacts they may cause to other organisms.
One example of a lasting major environmental impact of the spill to other species from exposure to crude oil is pelagic fish cardiac and swim performance impairment which, in turn, has been found to lead to the inability of embryonic development. Mahi-mahi embryos obtained from the University of Miami Experimental Hatchery and yellow fin tuna embryos obtained from the Inter-American Tropical Tuna Commission’s Achotines Laboratory were collected as experimental specimen and exposed to different dilutions of crude oil collected from the Deepwater Horizon Oil Spill site, as well as varying levels of ultraviolet radiation (UV) exposure, for 96 hours in a pelagic embryo-larval exposure chamber (PELEC). Mahi-mahi specimens exposed to higher levels of UV radiation were found to have a nine-fold increase in toxicity from Deepwater Horizon crude oil increasing stress levels within the fish. Yellow fin tuna survival rates were found to be significantly higher in the PELEC system than in the agitated system, meaning their survival rate decreased by a measure of 20% when exposed to crude oil and UV radiation (Steiglitz). Events such as the Deepwater Horizon oil spill can challenge pelagic fish, especially embryos and their ability to develop correctly and survive. Thus, this research provides ways in which we can begin to predict the extreme environmental conditions species would face in future oil spills, as well as examine how remnants of oil preserved in sediments may affect spawning grounds among certain species.
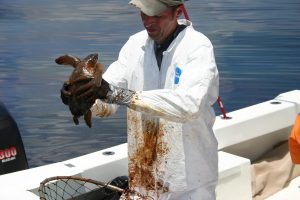
Kemp’s Ridley sea turtle (Lepidochelys kempii) covered in crude oil
(Source: http://www.noaanews.noaa.gov/stories2015/ 20150504-noaa-announces-new-deepwater-horizon-oil-spill-searchable-database-web-tool.html).
Another example of the diverse and devastating impact that an oil spill can have can be found in northwest Florida, where the loggerhead turtle (Caretta caretta) has been found to have varying offspring densities in nests since the Deepwater Horizon spill in 2010. Using a before-after, control-impact statistical model, researchers from the US Fish and Wildlife Service and Florida Fish and Wildlife Conservation Commission examined the historical records of loggerhead turtle nest densities and compared them to nest densities after 2010. They found that loggerhead nest densities in 2010 were reduced by 43.7% following the Deepwater Horizon oil spill and approximately 251 nests were decimated by crude oil and cleanup efforts, having a long-term impact on population sizes (Lauritsen). The drastic decline is due in part to the oil that entered “nearshore areas and washed onto beaches along the northern Gulf of Mexico shoreline during the summer of 2010, requiring extensive, disruptive activities to remove contaminated beach sand, oil, and debris” (Lauritsen). Nesting densities increased to normal rates in 2011 and 2012 suggesting some loggerhead sea turtles avoided mortality from oil saturation. Researchers later estimated that at least 65,000 sea turtles perished in 2010, likely exacerbated by oil contamination (Pallardy).
There are few places on earth as lovely and naturally beautiful as the Gulf of Mexico. From its sandy white beaches, coastal marshes and abundant estuaries, to its serene salt waters, the Gulf region is a critical environment that humans and countless animal species rely upon for food, shelter, and recreation. Sadly, since 2010 when the Deepwater Horizon spill event took place, there have been at least 234 additional oil spills here in the United States as of December 2017 (ITOPF). And while the immediate impact of a spill is unacceptable, the lasting legacy such as sediments that retain oil particles long after a spill occurs and its impact on range of species across the food chain from microorganisms to sea turtles to mahi-mahi and yellow fin tuna should concern all of us. As populations continue to grow, so too will energy needs and this, along with the constant threat from yet another oil spill and the long-term implications its pollution has on our environment, makes managing these risks, while also embracing and evolving to sustainable energy solutions, critical to nature and humans alike.
Works Cited
Frost, E. (2018, February 28). Gulf Oil Spill. Retrieved March 15, 2018, from http://ocean.si.edu/gulf-oil-spill
Huettel, M., Overholt, W. A., Kostka, J. E., Hagan, C., Kaba, J., Wells, B., & Dudley, S. (2017, December 22). Degradation of Deepwater Horizon oil buried in a Florida beach influenced by tidal pumping. Retrieved March 13, 2018, from https://www.sciencedirect.com/science/article/pii/S0025326X17309037
ITOPF. (2017, December). Oil Tanker Spill Statistics 2017. Retrieved March 15, 2018, from http://www.itopf.com/knowledge-resources/data-statistics/statistics/
Lauritsen, A. M., Dixon, P. M., Cacela, D., Brost, B., Hardy, R., MacPherson, S. L., . . . Witherington, B. (2017, January 31). Impact of the Deepwater Horizon Oil Spill on Loggerhead Turtle Caretta caretta Nest Densities in Northwest Florida. Retrieved March 13, 2018, from http://www.int-res.com/articles/esr2017/33/n033p083.pdf
Pallardy, R. (2017, December 15). Deepwater Horizon oil spill of 2010. Retrieved March 15, 2018, from https://www.britannica.com/event/Deepwater-Horizon-oil-spill-of-2010
Stieglitz, J. D., Mager, E. M., Hoenig, R. H., Alloy, M., Esbaugh, A. J., Bodinier, C., . . . Grosell, M. (2016, July 22). A novel system for embryo-larval toxicity testing of pelagic fish: Applications for impact assessment of Deepwater Horizon crude oil. Retrieved March 13, 2018, from https://www.rsmas.miami.edu/users/grosell/PDFs/2016 Stieglitz et al.pdf&p=DevEx,5063.1