By Timothy Hogan, SRC Intern
In response to overfishing, scientifically-derived annual catch limits and other regulations were developed to protect many declining species. Despite this, some understudied organisms could not receive the same improvements, where minimum data and low resolution made their abundance relatively unclear. Catch limitations became relatively difficult to set, as the population trends of many species could not be analyzed with confidence. Recent studies have managed to develop approximate regulations despite the lack of data (Newman et al 2015). However, a lack of coordination and data review continues to leave gaps in our understanding of these organisms.
Skates, cartilaginous fish closely related to rays and sharks, are one of these data-poor species. Because of their low economic benefit, and the lack of understanding behind their aging and population dynamics, the aging and regulations behind these organisms become difficult to set with confidence (Miller et al 2009). As important predators that control the populations of many crabs, scallops, and other organisms on the seabed, they remain important to control and protect as some of the more data-rich species.
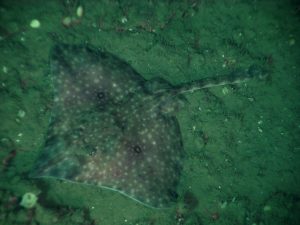
Figure 1: A long-nose skate found off the coast of Washington. While similar to rays, skates are generally distinguished from their relatives due to their longer snout, two dorsal fins along their thicker tail, and thorn-like scales along their middle back
The northeast skate complex, composed of the seven species of skates found along the Northwest Atlantic shelf system, remains to be relatively well-studied and monitored compared to some other skate groups. While recent reports state that all but one species are not experiencing overfishing (Miller et al 2009), the low resolution of the data makes these results more inconclusive. Regulations are therefore generally set on the life history of these species, since species size and growth rate are common indicators of an organism’s resilience to fishing pressures (Frisk et al 2001). Studies by Kelly and Hanson (2013) show that the maturity age of two particular species, the winter skate and the little skate, is relatively late compared to most other commercial fish. These types of organisms, known as K-strategists, are put under careful monitoring by many regulation groups, as they tend to be the most susceptible to overfishing. Figure 1 showcases the overall population declines that have been observed from these effects (Kelly and Hanson 2013). The majority of individuals are not able to survive to maturity from fishing pressures, causing overall population declines over time. While this may also simply be due to the low catch and landing rates of skates, it is still important to consider the potential population decline and lengthened recovery time.
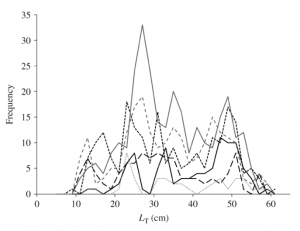
Figure 2: A frequency – size distribution of Little Skate specimens collected throughout various years. The solid gray line represents the year 2007, and shows a high peak below the age range of maturity, beginning at 32 cm. Samples from 2008 and 2009, the two dotted lines, are seen to be much lower. This could be due to a natural decrease from fishing pressures or a low catch number (Kelly and Hanson 2013)
In the past, skates were targeted for their fins, which would primarily be used as lobster bait and occasionally food (Cavanagh and Damon-Randall 2009). However, because their slow reproduction rates increased their vulnerability, possession and landings of skates have been significantly reduced since 2003. Prior to this, the population conditions of the skates have been relatively unmonitored, making it difficult to tell the population state. Current regulations placed based on new data can hopefully encourage population rebuilding of these organisms, leading to an eventual recovery.
A more prominent and unmeasured cause of skate depletion both historically and currently has been bycatch. Because they are found throughout the deep waters of the coast, they can be unintentionally caught by fishing equipment that collect organisms from the seabed. Notably, haddock, an important commercial fish found in the Northeast Coast of the United States, were caught using trawling nets. While these could catch the target species relatively effectively, it was infamous for also taking many other organisms from the seabed, including crabs, flounders, and skates (Beutel et al 2008). In times of high fishing for these haddock, major effects can be observed on a variety of other organisms. The barndoor skate has been eradicated from specific geographic areas due to being caught in fishing gear alone (Cavanagh et al 2009).
Because of its effects and historical damage, it is crucial to take bycatch into account when developing regulations. For most organisms, it is difficult to quantify unintentional catches because of a lack of data. Most data-poor species do not have the same level of enforcement as some of the more commercially important species. When collecting numbers for fishing regulations, most data is collected on shore from samples present on the ship. When caught as bycatch, many skates and other organisms are simply discarded into the water before. Unfortunately, this leaves the most prominent source of population damage difficult to be measured.
Even without the necessary data, a variety of efforts have been made to reduce the potential bycatch on rays as well as other organisms. New technology is continuously developed to reduce bycatch, including an Eliminator TrawlTM which reduced skate by catch from 33.4% to 1.4% of the weight of the target haddock, allowing a significant amount of preservation (Beutal et al 2008). New regulations and methods are continuing to be established to these data-poor species, allowing more accurate fish stocks and subsequent to be developed for these species (Newman et al 2015). However, many of these elements remain in early development, and still require a significant amount of data before they can be used effectively.
In order to improve regulations on skate fisheries, it is imperative to put more effort into learning about skate dynamics. While there is limited information on many of these aspects, using more data-limited assessments to analyze these species may be used to collect data, though any conclusions should be monitored often. In the future, it is important for regional management offices to coordinate and pool their data, while also creating a standard set of regulations to maintain these organisms in multiple locations. The current best practices for protecting skates and other fish with minimal data are relatively unknown, but given communication and extensive studies, it remains possible for these organisms to be better preserved and understood in the future.
Bibliography
Newman, D., Berkson, J., and Suatoni, L (2015). Current methods for setting catch limits for data-limited fish stocks in the United States. Fisheries Research, 164, 86-93.
Phillips, S.RM, Scott, F., and Ellis, J.R (2015). Having confidence in productivity susceptibility analyses: A method for underpinning scientific advice on skate stocks? Fisheries Research, 171, 87-100.
Beutel, D., Skrobe, L., Kathleen, C., Rhule Sr., P, Rhule Jr., P, O’Grady, J., and Knight, J (2008). Bycatch reduction in the Northeast USA directed haddock bottom trawl fishery. Fisheries Research, 94(2), 190-198.
Frisk, M.G., Miller, T.J., and Fogarty, M.J (2001). Estimation and analysis of biological parameters in elasmobranch fishes: a comparative life history study. Can. J. Fish. Aquat. Sci., 58, 969-981.
Kelly, J.T. and Hanson, J.M. Maturity, size at age and predatory-prey relationship of winter skate Leucoraja ocellata in the southern Gulf of St. Lawrence: potentially an undescribed endemic facing extirpation. Journal of Fish Biology, 82, 959.
Millers, T., Muller, R., O’Boyle, B., and Rosenberg, A. (2009). Report by the peer review panel for the Northeast data poor stocks working group. Woods Hole (MA): Northeast Fisheries Science Center. Report for the Data Poor Assessment Working Group.
Cavanagh, M.F., and Damon-Randall, K (2009). Status of the barn door skate (Dipturus laevis). National Marine Fisheries Service Report, Northeast Regional Office.
Bowley, S. (2008, April 12). A long-nose skate. Washington, Olympic Coast NMS. Retrieved March 17, from Wikimedia Commons: https://commons.wikimedia.org/wiki/File:Olympic_Coast_National_Marine_Sanctuary2008_Rajidae.jpg