By Brenna Bales, SRC intern
Popular opinion conjectures that sharks are always the dominant predator in their specific environments. The famous, terrorizing shot of the great white shark leaping out of the water with the unsuspecting seal in its jaws is iconic to Discovery Channel’s “Shark Week” highlight reel every year, boosting this notion of shark dominance. But what many don’t realize is that the seal is more capable than it may seem in those dramatized, slow-motion clips; In some cases, the role may be reversed.
Intraguild predation (IGP) is the killing or consuming of species that are also potential predators, a combination of both competitive and parasitic/predatory interactions (Polis et. al., 1989). What is so special about this type of relationship between two species? Fundamentally, the act of killing a predator and using it as a source of energy impacts more than just the two species directly involved. Instead, it affects other populations indirectly, and can stabilize or destabilize an environment in different ways. For example, population declines based on age differences can occur, as a species A adult will predate over a species B adult; However, a species A juvenile is preyed upon by a species B adult (Polis et. al., 1989; Figure 1). If there are less species A juveniles due to predation, then there will subsequently be less species A adults, thus reducing threats on species B at all ages. These changes trickle down the trophic levels, affecting species A and B prey items and their respective food sources, as changes in apex predator populations have cascading trophic effects (Myers et. al., 2007).
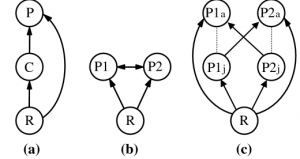
Figure 1: Three types of intraguild predation: (a) simple, (b) reciprocal, (c) reciprocal age-dependent.
https://openi.nlm.nih.gov/imgs/512/178/2853694/PMC2853694_442_2010_1575_Fig1_HTML.png
Overall, IGP can be age-, density-, and resource-dependent, disturbing established trophic and population dynamics such as above. There are two IGP descriptors, each with two categories: symmetry (asymmetrical/symmetrical) and age structure (important/relatively unimportant) (Polis et. al., 1989). We will first examine an asymmetrical IGP event. Observations by Chris Fallows in waters off Cape Point, South Africa led to the discovery of the predation of Cape fur seals (Arctocephalus pusillus pusillus) on blue sharks (Prionace glauca). This is the first evidence of asymmetric IGP on a mid-sized predatory shark by a pinniped (Fallows et. al., 2015). Therefore, these observations were exciting. The seal only consumed the viscera (main internal organs such as intestines, stomach, liver, etc.) after chasing and tossing the shark for several minutes (Figure 2). It proceeded to perform similar actions and kill 5 out of 10 sharks in the vicinity. This behavior is significant because in the past, the opposite interactions have been observed, in which adult blue sharks chased juvenile and adult Cape fur seals (Stewardson 1999). This behavior is similar to the situation between species A and B explained above; Although, in the end, not enough evidence is presented to strictly attribute age-dependency to the relationship between blue sharks and Cape fur seals.
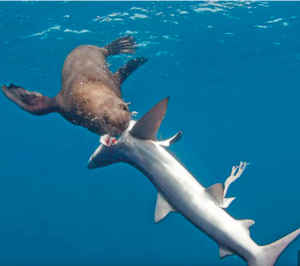
Figure 2: A Cape fur seal feeds on a blue shark. Taken from: Fallows, C., Benoît, H.P. and Hammerschlag, N., 2015. Intraguild predation and partial consumption of blue sharks Prionace glauca by Cape fur seals Arctocephalus pusillus pusillus. African Journal of Marine Science, 37(1), pp.125-128.
Another first for shark-pinniped IGP observations was an incident off the coast of New South Wales, Australia. An Australian fur seal (Arctocephalus pusillus doriferus) was observed feeding on an approximately 1.4-meter wobbegong shark (Orectolobus ornatus) (Allen and Huveneers, 2005). It was inferred that the incident was predatory due to the fact that the shark’s bodily condition would have appeared different had it been opportunistically ripped off a hook from a long-line, and rejection by a fisherman was unlikely. In addition, wobbegong sharks have never been found in the diet of any other predatory animal, although elasmobranchs such as the puffadder shyshark (Haploblepharus edwardsii) and the spiny dogfish (Squalus acanthias) have been (Allen and Huveneers, 2005). This supports the need to examine changing predatory roles in marine environments, as more than one threatened species at the top of the food chain can have serious conservation implications, and the removal of one species may have consequences not previously considered.
Switching from pinnipeds to cetaceans, recently popularized killer whale (Orcinus orca) and great white shark (Carcharadon carcharias) interactions have flipped the public’s vision of the great white as the ocean’s most fearsome predator. However, these interactions have been observed and officially recorded since the 1990’s around the Farallon Islands by researchers such as Peter Pyle and Scott Anderson. Both species consume pinniped prey, and the killer whales will occasionally consume the white sharks, clearly an example of IGP (Pyle et. al., 1999), and one that contradicts long-held beliefs.
There are two cases in which the same type of IGP interactions have different outcomes: when prey is abundant, and when it is not. If resources are abundant, competition will be low, and species A and B will, for the most part, coexist. However, when the situation is reversed and prey resources are limited, species A may begin to prey upon species B, thus becoming an IGP situation. The major sharks that are thought to prey on cetaceans are the white shark (Carcharadon carcharias), tiger shark (Galeocerdo cuvier), bull shark (Carcharhinus leucas), sixgill shark (Hexanchus griseus), sevengill shark (Notorynchus cepedianus), dusky shark (Carcharhinus obscurus), oceanic whitetip shark (Carcharhinus longimanus), shortfin mako shark (Isurus oxyrinchus), pacific sleeper shark (Somniosus microcephalus), greenland shark (Somniosus microcephalus), cookie-cutter shark (Isistius brasiliensis), and portugese dogfish (Centroscymnus coelolphis) (Heithaus 2001). However, reciprocal predation/consumption by cetaceans on these species is rare to non-existent, although aggression is often observed from both cetaceans and pinnipeds (Heithaus 2001; Stewardson and Brett, 2000; Kirkwood and Dickie, 2005).
In conclusion, unexpected intraguild predation between different species is dependent on a variety of environmental, opportunistic, and resource related variables, and expands beyond the marine environment (Polis et. al., 1989). As declines of elasmobranch, pinniped, and cetacean populations continue, lower trophic levels may or may not even be affected when other species subsequently become dominant predators, with only the upper trophic levels being affected. Nonetheless, these are important considerations to factor into an ecological assessment or examination, as climate and anthropogenic stressors mount on wild populations.
Literature cited:
Allen, S. and Huveneers, C. (2005). First record of an Australian fur seal (Arctocephalus pusillus doriferus) feeding on a wobbegong shark (Orectolobus ornatus). Proceedings of the Linnean Society of New South Wales 126, 95-97.
Bowen WD, Iverson SJ. 2013x. Methods of estimating marine mammal diets: a review of validation experiments and sources of bias and uncertainty. Marine Mammal Science 29: 719–754.
Fallows, C., Benoît, H.P. and Hammerschlag, N., 2015. Intraguild predation and partial consumption of blue sharks Prionace glauca by Cape fur seals Arctocephalus pusillus pusillus. African Journal of Marine Science, 37(1), pp.125-128.
Heithaus, M.R., 2001. Predator–prey and competitive interactions between sharks (order Selachii) and dolphins (suborder Odontoceti): a review. Journal of Zoology, 253(1), pp.53-68.
Kirkwood, R. and Dickie, J., 2005. Mobbing of a great white shark (Carcharodon carcharias) by adult male Australian fur seals (Arctocephalus pusillus doriferus). Marine mammal science, 21(2), pp.336-339.
Myers, R.A., Baum, J.K., Shepherd, T.D., Powers, S.P. and Peterson, C.H., 2007. Cascading effects of the loss of apex predatory sharks from a coastal ocean. Science, 315(5820), pp.1846-1850.
Polis, G.A., Myers, C.A. and Holt, R.D., 1989. The ecology and evolution of intraguild predation: potential competitors that eat each other. Annual review of ecology and systematics, 20(1), pp.297-330.
Pyle, P., Schramm, M. J., Keiper, C. & Anderson, S. D. (1999). Predation on a white shark (Carcharodon carcharias) by a killer whale (Orcinus orca) and a possible case of competitive displacement. Mar. Mamm. Sci. 15: 563±568.
Stewardson CL. 1999. Preliminary investigations of shark predation on Cape fur seals Arctocephalus pusillus pusillus from the Eastern Cape coast of South Africa. Transactions of the Royal Society of South Africa 54: 191–203.
Stewardson CL, Brett M. 2000. Aggressive behaviour of an adult male Cape fur seal (Arctocephalus pusillus pusillus) towards a great white shark (Carcharodon carcharias). African Zoology 35: 147–150.