Swimming and Diving Energetics of Dolphins Can Help Predict the Cost of Flight Response in Wild Odontocetes
By Chelsea Black, SRC MPS student
There are many occasions when high-speed swimming might be demanded by free-ranging marine mammals. This behavior will come at an energetic cost to the animal, which is why it is usually only performed when necessary for survival of the animal. Williams et al. (2017) demonstrates the physiological consequences of oceanic noise on diving mammals, in the hopes of providing a tool for predicting the biological significance of escape responses by cetaceans facing anthropogenic disturbances.
The physiological response of fleeing marine mammals has been challenging to study due to the difficulty of simultaneously measuring both metabolic rate and swimming behavior in free-ranging cetaceans like dolphins and whales. Studies performed in lab settings can provide invaluable information to answer these unknowns. In a study by Williams et al. (2017), the energetic cost of producing a swimming stroke by exercising and diving bottlenose dolphins was measured by calculating oxygen consumption and stroking kinematics of trained bottlenose dolphins (Tursiops truncatus) and one killer whale (Orcinus orca). The animals were housed in saltwater pools at Long Marine Laboratory in Santa Cruz, where they were trained to either voluntarily rest or exercise at various levels. To measure the energetic cost of diving, the dolphins were fitted with a submersible accelerometer recorder and performed three different experimental conditions: voluntary rest at the surface, rest while submerged, and submerged swimming and diving exercises. The results show little change in oxygen consumption between rest and routine swimming speeds, most likely due to the animal’s exceptional streamlined bodies that minimize hydrodynamic resistance. In contrast, there was a marked increase in oxygen consumption during higher level performances such as high-speed swimming, which affected the total amount of oxygen utilized during the dive (Williams et al., 2017).
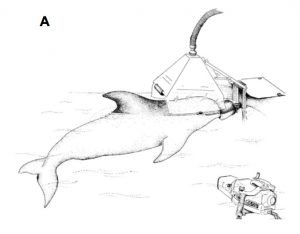
Figure 1: Dolphins breathe into a metabolic hood to analyze respiration (Williams et al., 2017).
Diving mammals must balance both speed and the duration of breath-holds, with limited available oxygen stores to minimize their energetic costs (Williams et al., 2017). High-speed swimming, increased stroke frequencies and rapid ascent from depth are commonly reported for wild tagged cetaceans following exposure to noise (Todd et al., 1996; DeRuiter et al., 2013). This particular response to noise exposure has been suggested as a cause for many marine mammal strandings, but scientists are less certain about how these responses translate into physiological costs to the animal.
The cost of flight by odontocetes is likely more complicated than counting the number of swimming strokes during a dive, therefor, the gait of the animal must also be considered. After using the calculations gathered from dolphins, Williams et al. (2017) could test the energetic cost of a dive after exposure to anthropogenic noise in the Cuvier’s beaked whale (Ziphius cavirostris), a deep diving odontocete considered to be particularly sensitive to underwater noise. In a dive without noise exposure, the whale spent over four minutes gliding on descent, however, when exposed to noise disturbance the whale did not use this energy-saving swim style, which increased its energetic cost.
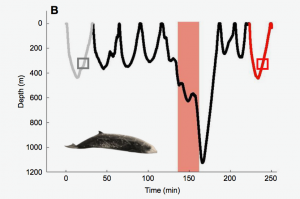
Figure 2: Behavioral response of Cuvier’s beaked whale to anthropogenic noise (Williams et al., 2017).
Overall, the beaked whale did not exceed its dive limit by reducing its depth and duration of the dive after a noise exposure while also increasing the use of energetically costly high-speed strokes. By using this combination, the whale was able to keep the proportion of available oxygen expended below the total amount available. Conversely, long and deep dives that exceeded one hour and 1000 m that occurred after sonar exposure, exceeded the oxygen stores. A common strategy for reducing energetic costs during these extreme dives was prolonged gliding during descent, which suggests that the role of swimming style is crucial in deep-diving species.
The data gathered from bottlenose dolphins and an orca provided a basis for applying the principals to wild marine mammals, illustrating the power of integrating energetics with swimming behavior and dive characteristics to assessing the impact of anthropogenic disturbances on cetaceans. While the oxygen stores and behavioral response will differ across species, this information will allow researchers to better predict the potential physiological consequences.
Works cited
DeRuiter, S. L., Southall, B. L., Calambokidis, J., Zimmer, W. M., Sadykova, D., Falcone, E. A., Friedlaender, A. S., Joseph, J. E., Moretti, D., Schorr, G.S. et al. (2013). First direct measurements of behavioural responses by Cuvier’s beaked whales to mid-frequency active sonar. Biol. Lett. 9, 20130223.
Todd, S., Lien, J., Marques, F., Stevick, P. and Ketten, D. (1996). Behavioral effects of exposure to underwater explosions in humpback whales (Megaptera novaeangliae). Can. J. Zool. 74, 1661-1672.
Williams, T. M., Kendall, T. L., Richter, B. P., Ribeiro-French, C. R., John, J. S., Odell, K. L., … & Stamper, M. A. (2017). Swimming and diving energetics in dolphins: a stroke-by-stroke analysis for predicting the cost of flight responses in wild odontocetes. Journal of Experimental Biology, 220(6), 1135-1145.