A spatiotemporal long-term assessment on the ecological response of reef communities in a Caribbean marine protected area
By: Megan Ando, SRC intern.
Marine Protected Areas (MPAs) have played a large role in the maintenance and conservation of vital marine ecosystems and species, many of which are endangered due to anthropogenic and natural causes. They also provide an ideal setting in which to perform long-term monitoring studies in order to analyze trends in coral reef communities, which provide a vast amount of insight into an ecosystem’s resilience. A compiled study, carried out over the course of 11 years by Martínez-Rendis et al., set out to observe one of these MPAs in hopes of assessing both the spatial and temporal long-term trends of some of the coral reef community indicators, which can be essential when it comes to mitigating and adapting to the several consequences of ecological shifts (Ricart et al., 2018). Being carried out in Cozumel, Mexico, studies like this are vital due to the recently recorded rapid degradation of coral reefs that are proven to be so important for the well-being of our planet (Mora, Graham, & Nyström, 2016). There have only ever been a few studies carried out in the Caribbean that provide time sequences and indicators of these systems, so studies like this provide the scientific community, and the world, with a great array of knowledge concerning the resilience of such a natural protected area.
As previously mentioned, this study took place in Cozumel, Mexico along six different reefs, all within different “zones” contained in the Cozumel Reefs National Park (CRNP) (Figure 1). These zones vary in their restrictions regarding fishing, scuba-diving, cruise ships, and other tourist activities that attract so many people to this reef system from around the world. The sampling performed in this area was executed through the use of transects, along which coral species, fauna densities, and other important details about the surrounding area were all recorded. The aforementioned indicators were also taken note of, which included densities of fish species, densities of trophic groups, species richness for each trophic group, relative cover of all scleractinian coral, and the corresponding relative coverage of macroalgae. Each of these indicators were eventually used in order to identify fish trophic groups with trends pertaining to the CRNP coral reef ecosystem, each of which would then infer information about the overall resilience of the MPA for future ecological conservation implications (Martínez-Rendis et al 2019). Such trophic groups have been used in the past to describe how fishing pressures affect reef trophic dynamics (Darling & D’Agata, 2017). Statistical tests were performed with all of this data to conclude their results.
Overall, it was found that differences in fish species appeared to be associated with distributions of the dominant species over the reef system, being controlled ultimately by environmental dynamics (Díaz-Ruiz, Aguirre-León, & Arias-González, 1998). Also, the changes seen in the densities of the fish trophic groups were both temporal and spatial, suggesting that both natural effects, including storms and hurricanes, and anthropogenic events, meaning construction and coastal development, cause changes in the abundance of these reef communities. As far as coral cover, there appeared to be a direct relationship between coral cover and corresponding macroalgae cover (Figure 2). Such algae cover could be promoted by an increase in coastal sediment discharge or other cumulative anthropogenic effects, which has the potential to surround and kill healthy coral.
To conclude, this group drew key conclusions regarding ecological trends and relevant constructive information that can be used to restructure the MPA for its own benefit as well as the benefit of the natural reef systems and all living organisms that it supports. It provides motivation for the further exploration of proper management strategies that need to be in respect to the tourists as well as the marine organisms in order to better conserve this reef system for generations to come.
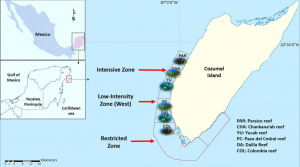
Figure 1: Map showing location of Cozumel off of the Mexican coastline, as well as the various reefs and zones being studied within the CRNP (Martínez-Rendis et al 2019).
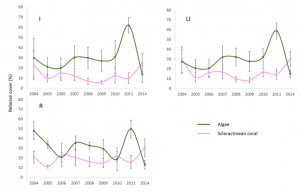
Figure 2: Graphic visual showing the direct trends in relative cover of scleractinian coral (purple) versus macroalgae (green) within the CRNP (Martínez-Rendis et al 2019).
Works cited
Darling, E. S., & D’agata, S. (2017). Coral. Reefs: Fishing for Sustainability. Current Biology.
Díaz-Ruiz, S., Aguirre-León, A., & Arias-González, J. E. (1998). Habitat interdependence in coral reef ecosystems: A case study in a Mexican Caribbean reef. Aquatic Ecosystem Health & Management, 1, 387–397.
Martínez-Rendis, Abigail, et al. (2019). A spatio-temporal long-term assessment on the ecological response of reef communities in a caribbean marine protected area. Aquatic Conservation: Marine and Freshwater Ecosystems, 30, 2, 273–289.
Mora, C., Graham, N. A. J., & Nyström, M. (2016). Ecological limitations to the resilience of coral reefs. Coral Reefs, 35, 1271–1280.
Ricart, A. M., García, M., Weitzmann, B., Linares, C., Hereu, B., & Ballesteros, E. (2018). Long-term shifts in the north western Mediterranean coastal seascape: The habitat-forming seaweed Codium vermilara. Marine Pollution Bulletin, 127, 334–341.